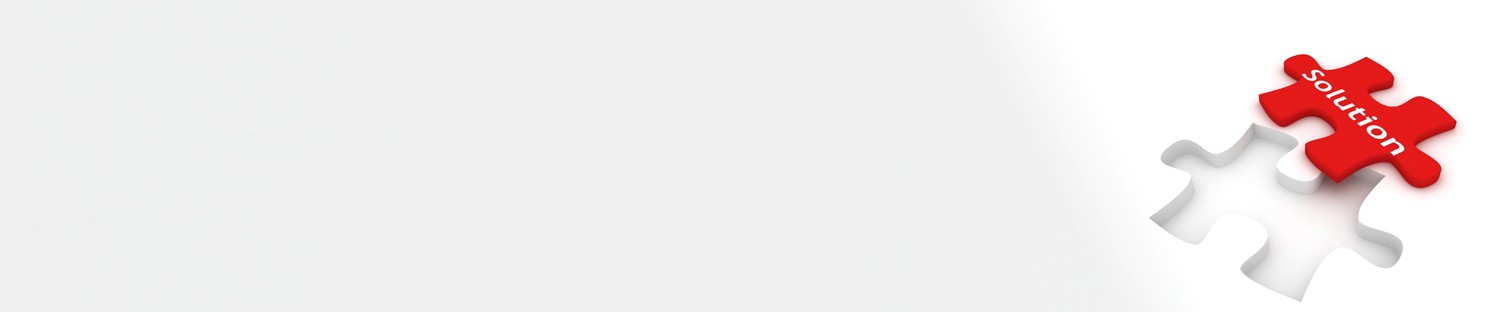
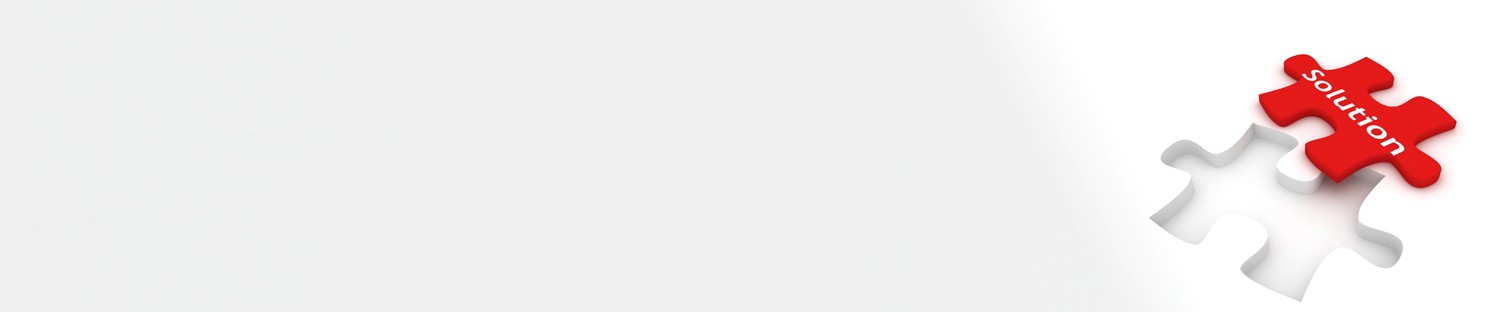
This Technology Brief examines the new IEEE Std 802.3bs-2017 standard for 400 Gbps and 200 Gbps Ethernet. The new standard continues the rapid development of Ethernet to accommodate the increasing bandwidth demands of cloud data centers. The current 400 Gbps specifications cover only fiber optic media and have pushed the limits of optical lane speeds and the number of parallel fibers in a link. As with most new Ethernet standards, a number of new transceiver form-factors, connectors, and cables have been developed to accommodate the new 400 Gbps standard. This new era of Ethernet innovation will no doubt cause a surge in new development that will last some time into the future.
With a 50 Gbps lane rate being the fundamental basis of reaching 400 Gbps, the first major decision was to change the signal encoding scheme. Up till now, all Ethernet standards have used simple 2-level Non-Return-to-Zero (NRZ) method for encoding a binary data stream into a transmittable electrical signal. To attain a higher lane data rate, an encoding scheme known as 4-Level Pulse Amplitude Modulation (PAM4) needed to be used, which effectively doubles the amount of data transmitted in the same amount of time.
If you think of binary data represented by a signal with two voltages, one voltage for a “0” and the other voltage for a “1”, then this describes the NRZ encoding method. For PAM4 encoding, the signal has four voltage levels, which encodes two binary bits per voltage level. A method known as “Gray coding” combines the most significant bit (MSB) and least significant bit (LSB) pairs in a data stream into one of the four voltage levels. Gray coding helps to reduce the bit errors in the signal caused by voltage amplitude noise. It is easy to see how with two data bits mapped to one voltage level, double the information can be transmitted in the same amount of time.
Name | Medium | Tx Fibers | Lanes | Reach | Encoding |
400GBASE-SR16 | MMF | 16 | 16 x 25 Gbps | 70 m (OM3)100 m (OM4) | NRZ |
400GBASE-DR4 | SMF | 4 | 4 x 100 Gbps | 500 m | PAM4 |
400GBASE-FR8 | SMF | 1 | 8 x 50 Gbps (WDM) | 2 km | PAM4 |
400GBASE-LR8 | SMF | 1 | 8 x 50 Gbps (WDM) | 10 km | PAM4 |
200GBASE-DR4 | SMF | 4 | 4 x 50 Gbps | 500 m | PAM4 |
200GBASE-FR4 | SMF | 1 | 4 x 50 Gbps (WDM) | 2 km | PAM4 |
200GBASE-LR4 | SMF | 1 | 4 x 50 Gbps (WDM) | 10 km | PAM4 |
Driven by the increasing demands of cloud data centers, the evolution of Ethernet standards has rapidly reached an incredible data rate of 400 Gbps. Using new encoding schemes and higher signaling rates, the 400 Gbps Ethernet standard marks a significant break from past standards and sets a path towards even faster speeds in the future. The new 400 Gbps and 200 Gbps standards include multimode and single-mode optical fiber options running from 70 m up to 10 km. The standards cover various cost-effective short-range and long-range applications and have been supported by a number of new transceiver form-factors, cables, and connectors.
As new 400 Gbps equipment becomes available, you can expect to see it quickly deployed in data center, carrier access, and service provider networks worldwide. The much-needed extra bandwidth will provide a boost as network traffic continues to increase year-on-year. However, Ethernet development will not stop there. New Ethernet working groups are already striving for higher speeds and more economical, compact form-factors that will keep pace with future industry demands.